Laboratory of synaptic physiology
Our research
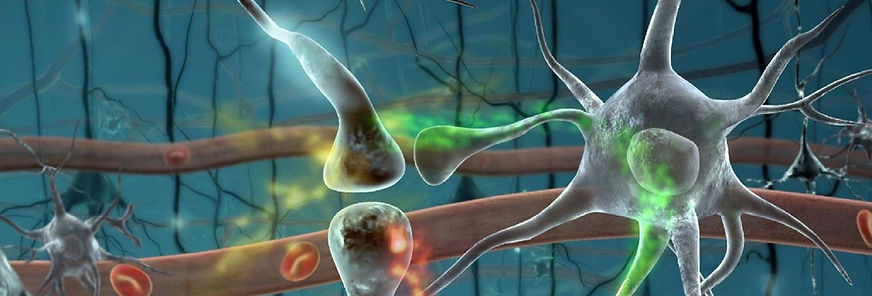
Our group aims to unravel mechanisms of synaptic computation in the mammalian brain and translate the scientific results to the clinic to combat diseases of the nervous system. The synapse consists of a presynaptic axon terminal and a postsynaptic structure that is often positioned in dendrites. Because axons and dendrites in the mammalian brain often have a diameter of less than 1 µm and are difficult to access, analysis of synaptic signaling in these thin structures represents a long-standing technical challenge in neuroscience. We use a combination of cutting edge electrophysiological, optical and computational tools to study synaptic computation in dendrites and axons of cortical neurons.
Currently, our group is focusing on two lines of research:
Synaptic computation of inhibitory interneurons
GABAergic interneurons provide inhibitory control of brain activity. A functional hallmark of several types of cortical interneurons is speed. To exert their special functions, these inhibitory neurons are capable of translating an input signal at their dendrites into an output signal from their axon terminals within less than one millisecond. This high speed of input-output computation enables the interneuron to play a key role in fast neural oscillations that contribute to a wide range of important functions in the brain. To reveal the design principle of the fast signaling, we use parvalbumin-expressing basket cells (PV+-BCs) in the rodent hippocampus as our model system to address following questions:
1. How are synaptic input signals integrated in interneuron dendrites?
We have studied how dendrites of interneurons process synaptic inputs. With the state-of-art confocal guided subcellular patch-clamp technique, we demonstrated that the functional properties of PV+-BC dendrites are remarkably different from those of the widely studied excitatory neuron dendrites (Hu et al., 2010; Nörenberg et al., 2010). The interneuron-specific dendritic signaling makes a major contribution to the fast synaptic computation at the input stage.
Hu, H., Martina, M., and Jonas, P. (2010). Dendritic mechanisms underlying rapid synaptic activation of fast-spiking hippocampal interneurons. Science, 327: 52–58.
Nörenberg, A., Hu, H., Vida, I., Bartos, M., Jonas, P. (2010). Distinct nonuniform cable properties optimize rapid and efficient activation of fast-spiking GABAergic interneurons. Proc Natl Acad Sci U S A, 107: 894-899.
2. How do interneuron axons produce synaptic output signals at high speed?
PV+-BCs play a key role in neural oscillations at high frequencies. To produce spatial coherence, individual PV+-BCs produce a widespread inhibitory synaptic output signal to control the activity of the surrounding network. By performing patch-clamp recordings directly from the thin axon of PV+-BCs, we have for the first time demonstrated that a high density of voltage-gated Na+ channels in the interneurons axon enables action potentials to propagate with high speed and reliability (Hu and Jonas, 2014). The fast action potential propagation provides each PV+-BC with a mechanism to synchronize the release of neurotransmitter (GABA) from thousands of axon terminals distributed over its extensive axonal arbor. Currently, we are investigating the specific mechanisms that couple fast action potential signaling to the release of GABA from PV+-BC axon terminals with high speed.
Hu, H. and Jonas, P. (2014). A supercritical density of Na+ channels ensures fast signaling in GABAergic interneuron axons. Nat Neurosci, 17: 686–693.
3. How does the fast interneuron signaling contribute to sensory processing in the cortex?
To study subcellular mechanisms during natural behavior, we use the rodent barrel cortex as a model system. This part of the somatosensory cortex processes tactile information coming from the whiskers that rodents use to gather information about their environment. By using electrophysiological, anatomical, and molecular tools, we aim to identify the role of fast signaling of cortical interneurons in sensory processing. With this knowledge in our hands, we will manipulate dendritic and axonal signaling in cortical interneurons with molecular tools and study how such manipulations modulate sensory processing in vivo.
4. How is the interneuron fast signaling impaired in diseases?
Being part of the medical faculty at the University of Oslo, a major goal of our research is to improve public health. We are focusing on the Dravet syndrome, which is caused by deficits of the SCN1a gene. The SCN1a gene encodes the voltage-gated Na+ channels that are highly expressed in interneuron axons. Mutations of this gene impair inhibitory interneuron functions in the brain and produce epilepsy in early childhood. By collaborating with Dr. Ethan Goldberg at the University of Pennsylvania, we take advantage of our expertise in subcellular electrophysiology to gain a better understanding of interneuron axonal dysfunctions in the Dravet syndrome.
Dendritic integration in hippocampal pyramidal neurons
Hippocampal pyramidal neurons play a key role in learning and memory. To understand the cellular mechanism of hippocampal memory representation, we study the rules of synaptic integration and synaptic plasticity in pyramidal neuron dendrites (Hu et al., 2009; Kim et al., 2012). The highly efficient synaptic activation of hippocampal CA3 pyramidal neurons by their recurrent collateral connections is thought to be instrumental for retrieving a full memory from a partial cue. By performing patch-clamp recording from CA3 pyramidal neuron dendrites, we found that recurrent synaptic inputs are strongly coupled to action potential outputs by dendritic Na+ channels. We also demonstrated that active action potential backpropagation in CA3 pyramidal neuron dendrites, which plays a key role in the Hebbian-form associative synaptic learning in these nerve cells, depends on dendritic Na+ channels. These results suggest that dendritic Na+ channels of CA3 pyramidal neurons may have important functions in hippocampal memory formation and retrieval. We have also demonstrated that specialized ion channels in CA1 pyramidal neuron dendrites amplify oscillating inputs at theta frequencies (Hu et al., 2009). As a continuum of this research, we want to determine dendritic mechanisms of how neurons are entrained by neuronal oscillations.
Kim, S., Guzman, S.J., Hu, H., Jonas, P. (2012) Active dendrites support efficient initiation of dendritic spikes in hippocampal CA3 pyramidal neurons. Nat Neurosci, 15: 600-606.
Hu, H., Vervaeke, K., Graham, L.J., Storm, J.F. (2009) Complementary theta resonance filtering by two spatially segregated mechanisms in CA1 hippocampal pyramidal neurons. J Neurosci., 29: 14472-14483.
Our research is supported by:
The Norwegian Reserach Council
The University of Oslo
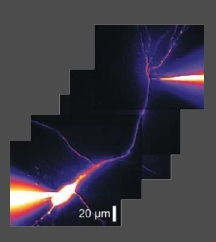
Figure 1. Confocal targeted patch-clamp recording from an interneuron dendrite. Data from Hu H et al. Science, 2010.